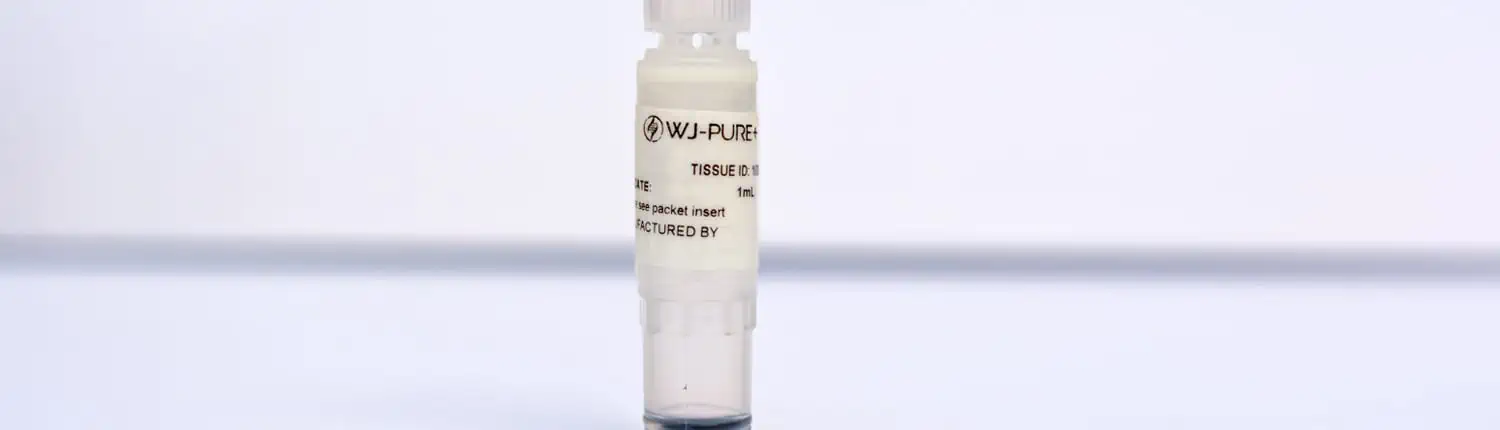
Essentially, all of the cells of the developing embryo are stem cells
First things first…. Embryonic stem cells that are used for medical treatments are NOT part of a developing fetus or baby.
Rather these cells are derived from other embryonic tissues such as placenta, umbilical cord, and amniotic fluid. These tissues are routinely collected during the birth of a baby and destroyed (incinerated) immediately following the birth.
From a clinical perspective, fetal stem cells are NOT suitable for any therapeutic use, because they are very unstable and not safe for transplanting into patients.
The 1st week of human embryo development. All of the cells are stem cells.
Embryonic stem cells are derived from embryos before the 2nd week of development long before the developing embryo has transitioned to becoming a Fetus. During these first two weeks, essentially all of the cells of the embryo are stem cells, in that they have not differentiated into cells with specialized functions.
Typically, these types of stem cells are derived from embryos that are created in laboratory conditions, not harvested directly from a human mother. In other words, a human egg has been harvested from a woman and fertilized with a human sperm in vitro (in a laboratory). This usually takes place in an in vitro fertilization clinic – and then donated for stem cell research purposes.
First, let’s dispel the misconception that developing fetuses (babies) are necessarily hurt, killed, or involved in any way with “embryonic stem cells”. They are NOT.
We are NOT referring to “fetal” stem cells that are harvested from a developing fetus, rather we are referring to stem cells derived from other embryonic tissues (not fetal) that are harvested after the baby is born.
These include placenta, umbilical tissues, umbilical cord blood and amniotic fluid. All these tissues are routinely destroyed after the baby is born and thus harvesting these tissues for research or medical treatments pose no ethical challenges at all regarding the life of the associated baby.
With the consent of the birthing mother, these tissues are professionally collected and taken to a specialized laboratory where the sought-after cells are extracted and stored. Of course, before these stem cells can be utilized, they must undergo comprehensive biological testing to ensure that the cells are free of disease, infections and genetic mutations. The parents of these cells are also tested and their health status validated.
Where serious ethical issues are encountered, are tissues harvested from frozen embryos that were created from in-vitro fertilization procedures.
In a nutshell, when a couple are not able to conceive a baby through natural intercourse, sperm and eggs can be collected, and fertilization can be induced in a laboratory, resulting in a nascent developing embryo. These embryos, which also contain a potential fetus, are frozen awaiting surgical implantation into the mothers’ uterus.
Once a pregnancy has reached viability, any remaining frozen embryos are no longer needed, and many medical research labs have wanted to use these frozen embryos for research. The challenge is that these frozen embryos contain the potential of a viable human life.
Growing stem cells in a cell culture
The technique of growing embryonic stem cells in the laboratory is referred to as cell culture.
Human embryonic stem cells (hESCs) are grown by harvesting the cells derived from an early stage preimplantation embryo (a very young embryo that if present in a human mother would not yet be implanted in her uterus).
These cells are grown in a special laboratory dish that contains a nutrient broth known as culture medium.
Once the cells have taken hold and are surviving they can be removed and placed into several additional culture dishes. The process is called sub-culturing the cells and can be repeated many times over many weeks and months.
Each cycle of sub-culturing the cells is referred to as a passage, and is a way that a few original stem cells can be “expanded” into many generations and millions of stem cells and are referred to as an embryonic stem cell line.
During the process of generating lines of embryonic stem cells in laboratory conditions, it is important to test the cells to see if they exhibit the basic properties or “characteristics” of stems cells. This process is called “characterization”.
Though this process has not been standardized throughout the cell-biology industry, the following are some of the tests that are commonly performed:
Healthy, undifferentiated stem cells under a microscope
Testing the cells’ ability for Self-Renewal. This is accomplished simply by growing and sub-culturing the stem cells for extended periods of time and evaluating their rate of growth.
At each stage, the stem cells can also be inspected through a microscope to ensure that they look healthy and remain undifferentiated.
Testing for the presence of specific Gene Transcription factors. Let me explain.
Every cell is constantly activating and deactivating specific genes which are the templates for the creation of different proteins. For instance, when we eat sugar, cells in our pancreas will create the protein insulin which is essential in regulating our blood sugar levels. To do this, certain transcription factors that turn-on the transcription of the insulin gene will be made. Conversely, when our sugar levels are low and we don’t need insulin any more, transcription factors will be made that inhibit the transcription of the insulin gene.
Basically transcription factors are what turn-on or turn-off the transcription of genes.
When stem cells are in an undifferentiated state, they produce certain transcription factors; specifically the “Oct-4” and “Nanog” transcription factors. Apparently, without these factors, the cells will start going down the path of differentiation. Therefore to establish that the cells we are growing are true undifferentiated stem cells, the transcription factors “Oct-4” and “Nanog” must be present.
All cells have specific membrane cell-surface-markers that are characteristic for that kind of cell.
Thus we can test for the presence of cell-surface-markers that are typical of stem cells.
Another important determination is to test for the cells durability. Can the stem cells be frozen and thawed and still remain viable.
The hierarchy of stem cells : We can test the cells to make sure they are in face pluripotent
Testing to see if the stem cells are truly pluripotent… meaning that they can differentiate into any kind of adult cell. This can be accomplished by allowing the cells to naturally and spontaneously differentiate, or by stimulating them by inducing them into differentiation.
Some labs even inject the stem cells into laboratory mice to see what kind of tissues might grow in the injected area. As you recall from the previous article, truly pluripotent stem cells can differentiate into any one of three fundamental cell types, (germ layers), endoderm, mesoderm and ectoderm cells.
As the embryo progresses through its development, stem cells will “differentiate” into fundamental “lines” of stem cells from which the entire human body is derived.
The most important aspect of this question and its answer is first to understand that every cell in our body, in every organ and every tissue, was created by a parent stem cell. At conception every cell in the embryo that eventually becomes a fetus and thereafter a baby, is a stem cell.
As the embryo progresses through its development, stem cells will “differentiate” into fundamental “lines” of stem cells from which the entire human body is derived.
Every adult tissue and organ has some stem cells that are responsible for replacing aging cells in that specific organ.
Therefore, from a strictly scientific perspective, the answer to this question is: stem cells represent every “line” of cells that comprise the entire human body.
From a more practical perspective, is the simple knowledge that every adult tissue and organ in a human body, has some stem cells that are responsible for replacing aging cells in that specific organ. Thus, for example, as a liver cell ages, and becomes less functional, it is programed to self-destruct (“apoptosis”) and is replaced by a new liver cell that is created by a local liver stem-cell.
Thus, the organs and tissues that have the greatest regenerative capacity are those that have the greatest percentage and most active stem cells.
Perhaps an even better question to ask is “how do we induce stem cells to differentiate into the exact tissue or organ we need”?
Let me explain. Obviously, the holy grail of regenerative medicine and stem cell therapy would be to grow a new organ – let’s say a liver – for a patient who has a diseased liver. In such a world, any damaged or diseased organ could simply be replaced by a new young organ generated right from the patient’s own stem cells.
Researchers are looking for the “magic recipe” for inducing a stem cell to differentiate into the cells we need or want
The hope is that by changing the composition of the nutrient base in which the cells are cultivated, or by adding certain transcription factors, or by using any number of chemical, biochemical and electronic elements, we might find the correct “recipe” for inducing a stem cell to differentiate into the cells we need or want.
Though we have discovered some basic protocols for limited induction of stem cells into specific organ tissues, we are far from growing a complete and viable human organ.
To date our best hope is focusing on developing a specific cell type and not the entire organ. For example the cells that produce insulin within a pancreas, but not the entire pancreas.
As it relates to medicine, embryonic stem cells are used in many areas of research. The most dramatic research is focused on areas where conventional approaches are woefully ineffective and offer little hope, and where stem cells are demonstrating significant potential and progress.
Examples include, neurological damage, neuromuscular diseases, nerve diseases and organ replacement. There are many articles and studies on the NIH website for the following conditions (see links).
Embryonic stem cells are used in a new medical science called “Regenerative Medicine” for many conditions characterized by degeneration. Below are the two most common uses of stem cell therapy. These treatments may or may not be available in the United States. Please see our FDA Disclaimer for more information.
The most common examples are degenerative joint problems, such as physical injury or arthritis.
Regenerative Medicine specialists around the world report that virtually all the joints in the body are good candidates for these amazing stem cells (click previous link to see the scientific studies on the NIH website). Most commonly in need are knees, hips, shoulders and spine that are damaged, poorly functioning and often very painful.
Generally, joint damage is concentrated in deteriorated ligaments, cartilage and bone that are needed for joints to function properly.
In cases like these, conventional medicine offers two primary therapeutic options, drugs to control the inflammation and pain, and/or surgery. Of course, as we all know, even the best pharmaceutical drugs have many deleterious if not dangerous side effects on the entire body, and surgeries are also fraught with many serious complications.
On the other hand, Regenerative Medicine, with the help of Stem Cells offers a different solution. Instead of attempting to repair joints by using invasive surgeries or disruptive biochemistry (drugs), Regenerative Medicine specialists around the world report that treatments using stem cells heal the damage tissues by causing them to regenerate naturally.
A well-known example of this was Kobe Bryant, the famous basketball star who saved his career by traveling to Germany to have stem cells injected into his deteriorating knees.
To add even more good news, Regenerative Medicine specialists around the world report that stem cell therapy has no negative side-effects and often gives the patient positive “side-effects” that are not even the focus of the therapy.
Bottom line, you might want stem cells for a bad knee, but every physiological system in your body can potentially benefit from them!
Some of the most devastating diseases that many people suffer from are autoimmune in nature… wherein the patient’s own immune system has malfunctioned and is attacking healthy tissue.
A few of the more common examples are Rheumatoid Arthritis, Lupus, Graves Disease (Thyroid) and even Multiple Sclerosis (MS).
In these cases, Stem Cells are infused into the body directly into veins, and sometimes by injections into muscles. As in orthopedic cases, Regenerative Medicine specialists around the world report the results are often amazing… not only arresting the progression of the disease, but actually reversing it, and healing the patient (click previous link to see the scientific studies on the NIH website)!
This last point is considered the most dramatic and positive impact that Regenerative Medicine and stem cells have on human health and medical science. Whereas conventional therapies are focused on “managing” and controlling the ongoing deterioration of a disease, studies show that Stem Cell therapy can often reverse and heal a disease.
AMA Regenerative Medicine & Skincare | 1570 Brookhollow Dr., Santa Ana, CA 92705 | 6310 San Vicente Blvd STE 285, Los Angeles, CA, 90048 Disclaimer: though everything on our website comes directly from reputable publications and scientific journals; and though thousands of these articles are available on official government websites (https://pubmed.ncbi.nlm.nih.gov), they have not been evaluated by the Food and Drug Administration and the FDA has not certified, endorsed or approved any of the scientific findings as methods of treating or diagnosing any diseases or illnesses.